Oct 2004: Data Summary Image
Apr 2001: Full Proposal
Apr
2002: Fraser River Plume Image
Oct 2002: Poster Produced for
EPOC and PICES meetings 2002
Oct 2002: The Great Flurometer
Scaling mystery
Feb 2004: Ocean Sciences
Poster: Revisiting the timing of the spring bloom in the SoG
Feb 2004: Ocean Sciences
Poster: Assessing surface water properties and chlorophyll
concentrations with ferry-based instruments
|
Summary Figure of Data to
October 2004
|
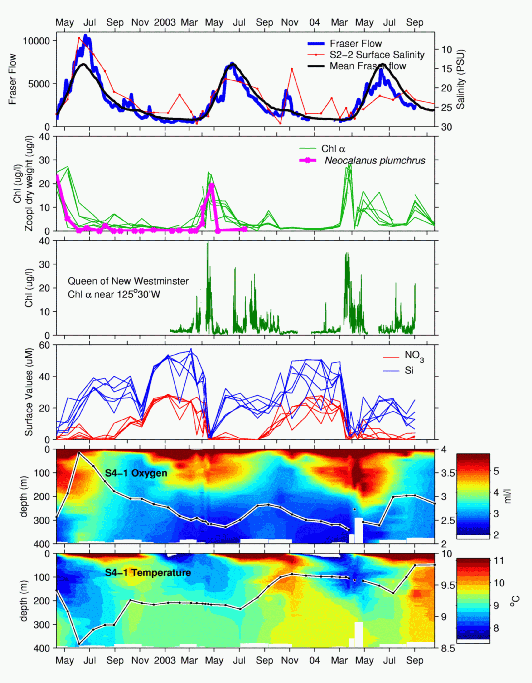
|
Full Proposal:
SECTION 1 - INTRODUCTION &
OBJECTIVES:
The Strait of Georgia is a highly productive, semi-enclosed, marine
ecosystem located between Vancouver Island and mainland British
Columbia (Fig. 1). In recent years the Strait has undergone
considerable changes, many of which are tied to the rapid population
growth in the lower mainland of British Columbia (by nearly 13% between
1991-1996). These changes have included increased usage of the Strait
for both commercial (e.g. fishing and shipping) and recreational
purposes (e.g. boating and sport-fishing), reduced air-quality, as well
as increases in sewage and other effluent from the greater Vancouver
region. There have also been significant changes in the marine
ecosystem of the Strait of Georgia. Surface water temperatures have
warmed by about 1ºC since the 1960's [2]. Certain fish species
(e.g. lingcod and certain rockfish) have been fished virtually to local
extinction. Increased occurrences of red tides and high fecal coliform
counts have led to more frequent shellfish harvesting closures and
raised concerns in the aquaculture industry. Plankton dynamics have
also changed, with key species now arriving at least a month earlier
than they did historically. However, it was the highly visible collapse
of the Strait of Georgia salmon stocks in the late 1990's, in what had
been one of the most productive salmon nursery grounds in the NE
Pacific, that finally served as the catalyst to raise public awareness
of the "State of the Strait". In response, several scientific and
community-based initiatives have been launched. The largest of these
projects, the Georgia Basin Ecosystem Initiative, involves a
partnership between several provincial and federal agencies to develop
action plans to improve air quality, to reduce water pollution, to
protect habitat and coastal biodiversity, and to support
community-based environmental initiatives within the Georgia Basin.
Fisheries and Oceans Canada (DFO) have also recently initiated a new
program (led by Richard Thomson at IOS) that aims to determine how
physical and biological processes in the SoG are linked to processes in
the adjoining Strait of Juan de Fuca and the continental shelf on the
west coast of Vancouver Island.
To date there has been little recognition of the
role played by natural physical variability in regulating biological
production in the Strait of Georgia (SoG hereafter). However, a growing
body of evidence suggests that changes in the productivity and
structure of this and other marine ecosystems are likely due to
interannual variability in the linkages between physical and biological
processes [1, 16, 17, 33, 34, 35]. Moreover, it appears that these
linkages may have recently been altered by climate change. Climate
affects the physical oceanography of the SoG through a number of
processes. ENSO oscillations and the North Pacific Decadal oscillation
can influence precipitation and air temperature (and therefore the
Fraser River outflow and timing) [1], poleward and equatorward winds
off the West Coast of Vancouver Island (and therefore the strength of
upwelling and the density/nutrient of deep flow through Juan de Fuca
[11]. Large scale climate oscillations also influence storm tracks and
the number and strength of storms in a particular region [25]. The
physical oceanography of the SoG affects the biology primarily through
nutrient availability, light availability, temperature effects on
physiological processes and temperature effects on predator migration.
Light availability to the phytoplankton is determined by the amount of
light reaching the surface of the water (determined by time of year and
cloud amounts), the depth of the mixed layer and clarity of the water.
Nutrient availability is determined by large scale nutrient fluxes into
and out of the estuary and the mixing of nutrients from deeper waters
into the mixed layer.
Biological-physical coupling has been the focus
of several high profile national and international oceanographic
projects (funded in part by NSERC) including OPEN, GLOBEC, JGOFS and
the newly funded SOLAS. However, it remains unclear whether observed
changes in ecosystem structure and productivity are driven primarily by
top-down or bottom-up control. In this study we will couple an
innovative field program with a series of models to determine the
dominant physical mechanisms underlying ecosystem structure. We will
use the SoG as our laboratory. In many respects the SoG is the ideal
environment in which to test the importance of "bottom-up" effects.
Effectively a semi-enclosed sea, the SoG has only limited exchange with
the open ocean via its southern end (Fig. 1) which is connected to the
Strait of Juan de Fuca (SJF hereafter). The net effect of this unique
geographic setting is that advective effects in the SoG are easy to
quantify, thereby making it much easier sample and model. Our request
is also quite timely, for two reasons. First, the new DFO program
mentioned was specifically designed to integrate and collaborate with
our proposed research. (Letters of support attached). Second, there are
signs that a major change in the SoG during 2000 (resulting in reduced
mortality rates of young coho salmon) may have been caused by a
particular combination of Fraser outflow and wind patterns which
created ideal conditions for strong primary and secondary productivity
(R.J. Beamish, DFO, pers. comm.) If, as some suggest, we are currently
on the cusp of entering a new regime then we are in a unique position
to follow this change as it develops.
THE PRODUCT: Our aim is to
develop a mechanistic understanding of the key links between physics
and biology in the SoG. This will enable us to generate a series of
"heuristic rules" linking variable physical oceanographic conditions
and biological oceanographic responses in the SoG. Our hope is that a
rule-based system of the sort proposed here can then be used by DFO to
evaluate the "oceanographic state" of the Strait for a given year. This
level of mechanistic knowledge of the links between biology and physics
is essential to implement an "ecosystem approach" to the management of
our renewable marine resources. Currently, there is no set of such
rules because no single environmental variable can explain the observed
variability. We envison the rules we propose to develop being along the
lines of: Strong winds, followed by a calm period and relatively
strong outflow will enhance the early summer primary productivity due
to diatoms, thereby producing a good "year". Similar rules will
outline the conditions leading to "average" and "poor" years. To
build these heuristic linkages we need to address four key issues: the
physical nutrient sources and sinks, the timing and magnitude of the
spring bloom, the summer primary productivity (and relative species
composition), and the timing and productivity of Neocalanus plumchrus,
the dominant copepod in the SoG. Each of these processes will be
affected by tides, winds and Fraser River input. Interannual variation
in the latter two will be partly determined by large scale climatic
variations.
OCEANOGRAPHIC SETTING:
Physical Oceanography: The SoG is a semi-enclosed marine
sea with significant fresh water input and strong stratification.
Stratification is especially strong near the Fraser River in a brackish
plume, mixed by both estuarine entrainment and surface wind mixing. The
plume is advected by winds and tides and its size varies by season with
the volume flux of the Fraser River. Freshet usually starts in April
and peaks in late June or early July with an approximately tenfold
increase in volume flux. There is considerable interannual variation
[9,15]. Winds in the SoG are generally lighter than on the outer coast
but strong storm winds do occur. As the strong winds are storm forced
there is considerable interannual variability. Most exchange with the
open ocean occurs via the SJF, and the overall flow can be considered a
classical two-layer estuarine exchange. Both the outgoing upper
brackish waters and the incoming deep ocean waters are significantly
modified by tidal mixing, both in the SJF and the islands at its
eastern end [15, 23] but layer transports are 5-20 times larger than
Fraser input.
Nutrients and Primary Production: The primary source of
nutrients in the SoG and SJF system is the deep estuarine inflow;
anthropogenic influences are considered relatively minor in comparison
[18]. The estuarine inflow traverses the continental shelf within the
deep Juan de Fuca Canyon and hence has its source in oceanic waters
well below the mixed layer. In consequence, observed nutrient
concentrations are always relatively high, varying from approx 25 uM in
winter to over 30 uM in summer [18]. Summer inflows are as high as 7000
tonnes N/day into SJF but much of this inflow is mixed upwards into
outflowing surface waters and only a small fraction (perhaps 10-30%)
actually enters the SoG [22]. Deep waters in the SoG are also high in
nutrients. Surface waters, however, are often very low in nutrients
during May-September [18].
Primary production in the SoG is believed to be limited by nitrogen
[10]. The Strait is light-limited during winter due to low light levels
and deeper mixed layers [10,17]. Winter SoG conditions are favourable
to nanoflagellates [29]. The increased light and stability of the
spring favour diatoms which usually bloom in succession (Thalassiosira
spp. followed by Chaetoceros spp.). Comprehensive cruises in 1991
[32,33,34] showed qualitatively that wind events can delay the spring
bloom. Stronger stratification in summer favours flagellates. However,
wind events can cause mixing and nutrient injection into the surface
layer. Summer blooms of various diatoms [27, 28] and dinoflagellate
species [30] also occur.
Zooplankton: The mesozooplankton community of the SoG is
similar to that of the oceanic NE Pacific and is dominated by the large
calanoid copepod Neocalanus plumchrus (Neocalanus, hereafter) [7].
Other species (particularly E. bungii, Calanus marshellae, C. pacificus
and Pseudocalanus minutus) may dominate to a lesser degree at other
times [10]. Numerous other small copepod species also inhabit the
surface waters over much of the year (e.g. Acartia spp., Centropages
sp., Chiridus gracialis, Metridia spp., Microcalanus pusillus,
Paracalanus parvus, Pseudocalanus spp.), however, the life history
patterns of these species are not well known [10].
LOGISTICAL APPROACH: We will
investigate the potential for bottom-up control in the SoG by
combining: (1) a targeted field program, (2) retrospective analysis,
and (3) coupled biological-physical modeling. Given the physical
proximity of the lead PI's (Allen/Dower/Pawlowicz), all three will
interact to attack each specific questions and the long term goal.
Travel costs have been budgeted to enhance collaboration with Li and
Denman.
Field Work (Dower, Pawlowicz, Harrison): Fieldwork will be
used to (a) determine the nutrient flux into/out of the SoG, (b)
monitor daily conditions within the SoG, and (c) obtain snapshots of
key physical and biological parameters a different times of the year.
In order to minimize costs for such an ambitious plan we propose a
variety of innovative approaches. First, work in progress by Pawlowicz
(NSERC-RG) should lead to a quantitative relationship between the
estuarine circulation and the Fraser River flow. Continuous deep
nutrient concentrations will be measured using a moored automated
nitrate sampler, now available from several companies. A continuous
record is desirable since deep inflows may "pulse'' with the
spring/neap cycle. Near-surface samples of nitrates, silicates, and
phosphates will be obtained during monthly surveys (see below). Second,
daily monitoring of conditions over a large part of the SoG will be
carried out using instrumented ferries (Fig. 1). In conjunction with J.
Gower (IOS), T/S/ Fluorescence will be measured over all tracks, with
nitrates measured over 1 path in the 2nd and 3rd years. Finally, we
propose using the CCG Hovercraft Siyay (based in Vancouver) to sample
at 8 stations throughout the SoG at roughly monthly intervals (for
hydrography, phytoplankton, zooplankton and nutrients). Dower and
Pawlowicz are already experimenting with this platform (NSERC-RG work).
The Siyay is also logistically convenient with great flexibility in
modifying sampling days as required for special occasions (or bad
weather) and with a cruising speed of 80 km/h is able to cover the
entire SoG.
Retrospective Analyses (Ingram, Pawlowicz, Allen): The SoG
is at the doorstep of two oceanographic institutes and two major
universities. Measurements have been made since the 1920 by various
investigators for various regions. We will assemble the available
historical physical and biological data and examine interannual
variability to investigate the temporal links between climate, physics
and biology in the SoG. Previous studies [24.5] have shown a positive
(warmer) link between strong ENSO events and sea surface temperature
along the B.C. coast. Extensive physical and chemical data, as well as
sporadic biological data, are available at the DFO laboratories in
British Columbia, UBC, UVIC, and the Marine Environmental Data Service.
Station data for meteorological forcing and river discharge will also
be obtained. Elsewhere [5.5], the importance of 10-15 year signals in
atmospheric/ runoff forcing was found to alter the sea surface
characteristics in the Gulf of St. Lawrence.
Modeling Approach (Allen, Dower, Denman, Walters): Several
approaches currently are used to model biological/physical systems. A
traditional approach (e.g. UB GLOBEC Georges Bank studies) is to couple
a relatively simple NPZ-type biological model to a fully 3D physical
model. Although this approach is attractive, in practice unknown or
neglected factors make results difficult to interpret in anything other
than general ways. Another approach is to design a relatively simple
"box-model" to parameterize the physics (with a fair degree of tuning)
which can be coupled to more detailed biological models [12, 16]. An
approach often used in open-ocean studies, [eg 5], is to couple a
simple biological model to a 1D mixed layer model. In this case it
becomes computationally easier to examine seasonal and interannual
changes, at the cost of tuning of the physical model to make it match
available observations. Obviously, a desirable goal would be to develop
a fully 3D physical model with complex biology. However, even were it
possible to create such a model of the SoG at this time, it is clear
that such models must be developed gradually, as the apex of a
hierarchy of simple models from which fundamental understanding will
flow. We will employ three complementary models. One will couple a
vertical mixed layer model (KPP) to a six compartment lower
tropic-layer model and a copepod life history model. The second will be
a dynamic mass balance model (Ecosim) that will be used to explore the
effects of bottom-up forcing on trophic interactions and population
dynamics of fish species. The third model (developed by our DFO
collaborator, M. Li) will use a full 3D physical model coupled to a
simpler biological model.
THE RESEARCH TEAM: Understanding
the biophysical coupling in the Strait of Georgia will require
expertise in a number of disciplines. We have assembled a team with a
broad and complementary skill set that brings together physicists and
biologists, modelers and observationalists. Collaboration will be
facilitated by our geographic proximity, as well as the fact that
several of us have already worked together and/or have co-supervised
students. Susan Allen (UBC) will have primary responsibility
for the coupled 1D biophysical model. Allen is a physical oceanographer
with experience in box-modeling coupled chemical-biological-physical
processes [12]. She was a member of the Canada GLOBEC Steering
Committee for 4 years and is one of Canada's representatives to PICES. John
Dower (UBC) will have primary responsibility for the biological
field measurements and the biology incorporated into the models. Dower
has extensive experience in bio-physical coupling (including
current-topography effects at seamounts, and turbulence effects on
zooplankton and larval fish) and is involved in spatially-explicit
modeling in coastal Newfoundland [24]. His group is currently studying
diapause and vertical migratory behaviour of Neocalanus in the SoG. Rich
Pawlowicz (UBC) will have primary responsibility for the physical
and tracer measurements. He has led major research missions in Haro
Strait and has focussed his recent research on the exchange and mixing
processes in the Juan de Fuca, Haro Strait, Georgia Strait system.
Currently he is completing fieldwork to determine the seasonal cycle of
nutrient fluxes including source/sink terms in Juan de Fuca and Haro
Strait. Grant Ingram, FRSC (UBC) is a physical oceanographer
who has worked on field studies of river plumes, coastal circulation
and mixing, and relationship between physical forcing and different
components of the marine ecosystem in the St. Lawrence estuary, Gulf of
St. Lawrence, and Hudson Bay. He was a principal investigator in the
Canadian JGOFS program, the OPEN NCE program, and various NSERC
strategic grants. Paul Harrison, FRSC (UBC) is an
internationally recognized phytoplankton physiologist. He was a member
of the Steering Committee for JGOFS Canada and is a lead-PI in SOLAS. Carl
Walters, FRSC (UBC) is a world leader in the development of
multi-species fisheries harvesting models and in fisheries
conservation. He was recently named one of 10 "Guardians of the Oceans"
by the Pew Charitable Trust. Ken Denman, FRSC (DFO/UVic) is an
internationally recognized expert in coupled biological-physical
models. He has systematically developed a 1D model for Ocean Station P
including iron limitation. He played a key role in JGOFS Canada and
GLOBEC Canada and was a member of the IGBP Global Climate Change Panel.
MANAGEMENT AND COLLABORATION:
Overall management of the project will be shared by Allen, Dower and
Pawlowicz with advice from Ingram. Pawlowicz and Dower will have
responsibility for the field program. Allen and Denman will have
primary direction of the modeling with input from Walters.
Retrospective analysis will be directed by Ingram. Harrison will lead
the analysis of nutrients and phytoplankton samples. Informal
collaboration will be greatly facilitated by our geographical proximity
and by our monthly cruises (travel money for visits to and from IOS is
budgeted). In addition, to ensure collaboration occurs between the
PI's, graduate students and the post-doc, we will hold a monthly
informal seminar/meeting series at UBC. In December of each year, we
will travel to IOS to give a scientific presentation followed by
smaller group meetings to discuss and coordinate our projects.
SCIENTIFIC APPROACH:
Physical Nutrient Sources and Sinks: Conceptually, we
envision the SoG as a bowl with only one major connection to the ocean
(SJF). Work in progress investigating SJF using a tracer inverse
technique [22, 23] will lead to estimates of transport and nutrient
flux through SJF into the SoG in 2000, and produce source/sink terms
for various parts of the SJF. Parameterizing transport as a function of
river inflow, and measuring nutrient concentrations in this proposed
work will allow us to (a) form budgets for the SoG (and hence estimate
"new production'') (b) investigate interannual variability, and (c)
provide advective flux terms necessary for the numerical modeling.
Spring Bloom Dynamics: The spring bloom timing is a
function of stratification due to river input, mixing due to wind and
grazing pressure due to Neocalanus [32]. What is the relative
importance of these three factors? Given a certain combination, what
will be the outcome? Ferry observations will give us the exact timing
of the blooms and their distribution across SoG. In addition the
surface salinity and temperature will give us an idea of stratification
(location of the Fraser plume). Neocalanus timing will be based on
observations (and preliminary analysis) from hovercraft observations.
With 3 field seasons planned it is anticipated that various different
situations will be observed using similar methodologies.
The 1D coupled model will be used to test the sensitivity to various
wind, fresh water and Neocalanus timing scenarios. The model will be
run Feb-Apr/May with initial conditions based on climatology for
February. Five wind, five fresh water and five Neocalanus
ontogenetic-timing scenarios will be combined to investigate the
sensitivity on the entire parameter space. The computational simplicity
of a 1D model will permit us to run the 125 scenarios multiple times,
if necessary. Scenarios for our three observation years and 1988, 1992
and 1993 [35] will be compared to field observations.
There is sufficient data available to run the Ecosim model from 1950 to
the present. When run in "hindcast mode" Ecosim can estimate the
primary productivity that would have been required to produce the
observed time-series of population dynamics at higher trophic levels.
Since not all primary production is available to the food chain that
leads to fish and other higher trophic levels, what this will really
provide is an estimate of the "target" amount of diatom-based primary
production that would have bee required in a given year. Thus, the
Ecosim model will be used to test the "heuristic rules" that we
generate using our 1D biological-physical coupled models. Given that we
have access to historical data on Fraser outflow and winds, we should
be able to see whether years that Ecosim suggests may have been "good"
years are also years when physical conditions were such that our 1D
biological-physical coupled model would have predicted high primary and
secondary production.
Summer Productivity: Summer productivity in the SoG is controlled
by nutrient availability to the surface euphotic zone. Yin et al.
(1997b) observed that summer productivity can be enhanced by wind
mixing or increased Fraser River outflow. They also observed a shallow
nutricline and postulated it was maintained by estuarine entrainment.
Quantitatively how important is wind? What types of wind event are
necessary to maintain production? Does the "windiness" of a summer
determine the type (large diatom or small flagellates) of phytoplankton
that dominates? Starting after the spring bloom (with a nutrient
depleted mixed layer) we will use the 1D biological-physical model
(without the full Neocalanus life history model) to investigate the
sensitivity to rate parameters in the two phytoplankton classes. The
biological model will have two phytoplankton classes; one representing
phytoplankton >10um (diatoms) and one representing phytoplankton
<10um. For reasonable physical and biological parameters we will
determine if both summers with mainly diatom growth and summers
dominated by smaller phytoplankton can exist. Because the actual
time-dependence of the wind (i.e. storms, etc) is expected to be
crucial, we will use actual wind data. Once again the ferry data will
be important for comparison. Phytoplankton biomass (as Chla), nutrient
concentrations and salinity/temperature (as proxy for stratification)
will be used. Phytoplankton species will be identified from samples
collected during the hovercraft surveys. We will use this data to
constrain the biological parameters using optimization (the simplicity
of the 1D model allows use of techniques like simulated annealing).
Neocalanus plumchrus Dynamics: Although Neocalanus is a key food
source for numerous fish species (notably several species of juvenile
salmon), it remains unclear what factors control interannual
variability in its biomass. The factors often cited as influencing
copepod growth rates are temperature and food (either quality or
quantity). However, given that Neocalanus is a strongly seasonal
species (spending only about 100 days in the surface waters and the
rest of the year at depth), it seems likely that its biomass during any
given summer is determined largely by the conditions encountered by the
population in the previous year.
The life history model that we will use was initially developed for the
Neocalanus population in the open NE Pacific (OSP). However, Neocalanus
behaviour in the SoG is different than at OSP; the overwintering period
is longer in the SoG, the time spent in the surface layer is shorter,
and the adults are larger than at OSP [21]. It has also been shown [2]
that the ontogenetic timing of the Neocalanus population in the SoG has
changed over the past 20 years (peak biomass now occurs one month
earlier than the historical mean). Unfortunately the SoG Neocalanus
population has received little attention in recent years, and so we
will begin by initializing our life history model with historical data
[7, 8]. We will update the model using the zooplankton data collected
monthly from our the eight stations in our hovercraft surveys (using
nets and an optical plankton counter), plus monthly data collected from
a single site in the central SoG since 1999 by Dower. We will then use
the life history model to explore to what extent changes in (i) spring
temperature (ii) overwintering temperature and (iii) food quantity or
quality in a given year affect the weight of overwintering females
(which determines their fecundity). Dower's lab is currently modeling
the effect of buoyancy on both the overwintering depth (of C5's) and
the vertical ascent rates (of naupliar stages) of Neocalanus in the
SoG.
MODEL DETAILS:
The KPP Model: The KPP model [14] has been chosen as the
mixed-layer model for the vertical 1D model. It is a non-local
turbulent diffusion model consistent with Monin-Obukov similarity
theory. The KPP model has been used in simulations of storms, diurnal
cycles, and seasonal weather patterns at OSP [13, 14]. It compares
favourably with observations and performs at least as well (in many
cases better) than other boundary layer models. We have a version at
UBC currently configured for Ocean Station P.
The Biological Model: This model will have six
compartments (1) Phytoplankton <10um (2) Phytoplankton >10um
(e.g. diatoms), (3) Microzooplankton (modeled after flagellates,
aloricate ciliates, and dinoflagellates) (4) New nitrogen (nitrate and
nitrite) (5) Regenerated nitrogen (ammonium and urea) and (6) Detritus
(includes PON, DON and heterotrophic bacteria). The phytoplankton
compartments will be partitioned so that the fraction of the biomass
present as species >10 um in size will increase with increasing
total biomass. Detritus maybe divided into several sub-compartments
based on size and regeneration rate. All compartments in the model will
be defined in units of nitrogen per cubic metre. Model fluxes will
determine the flow of nitrogen between theses compartments and will be
based on a suite of physiological processes: (1) Nutrient uptake
(controlled by ambient concentrations and light) (2) Ingestion (by both
micro and mesozooplankton) (3) Excretion (by both micro and
mesozooplankton) (4) Egestion (by both micro and mesozooplankton) (5)
Phytoplankton cell lysis (either auto- or viral lysis) (6) Zooplankton
mortality (and grazing by mesozooplankton & unmodeled higher
trophic predators) (7) Sinking losses and (8) Nutrient regeneration. We
will use a similar model (developed by N. Jeffery and S. Allen at UBC
for OSP, based on [5]) and adapt it to reflect conditions more
characteristic of the SoG.
Copepod Life History Model: A Neocalanus life cycle model has been
developed at UBC. The model includes weight dependent growth and
mortality, migration into and out of the upper ocean, molting between
stages and a stage distributed population. The total population is
divided into cohorts: cohorts share a common arrival time, maturity
distribution and weight distribution. The maturity distribution is
assumed Gaussian, and the mean and variance have prescribed evolutions
based on field observations of stage durations, however temperature
dependence will be included. For each day, maturity defines the
fraction of each cohort in the mixed layer, in a given stage, and in
diapause. Physiological processes, and thus stage biomass, molting
weights, and grazing, depend on the mean weight of each cohort and
other environmental cues such as predator and prey concentration and
temperature. The model solves a first order coupled nonlinear
differential equation for each mean cohort weight.
Ecosim Model: Walters has begun developing an Ecosim model for the
SoG. Ecosim is a dynamic simulation model which is an extension of
Ecopath, a well-known dynamic mass balance model [31] used to examine
trophic interactions and population dynamics due to either top-down
(e.g. fishing or predator-prey interactions) or bottom-up effects (e.g.
variability in primary production). The SoG Ecopath model contains 27
compartments, ranging from phytoplankton and detritus all the way up to
apex predators such as large fish (e.g. salmon and hake) and marine
mammals (e.g. seals and orcas). The Ecosim model is initialized using
historical catch statistics, abundance indices and dietary studies, in
combination with estimates of rate processes (e.g. growth and feeding
rates) drawn from literature sources. The model can then be used to
predict how higher trophic levels may be affected by changes in primary
productivity or, as we propose here, to reconstruct the primary and
secondary production necessary to have produced a given biomass
configuration of higher trophic levels.
FIELD WORK DETAILS: The first
component of our field work will make use of the Canadian Coast Guard's
high-speed hovercraft, the Siyay, to conduct 2-day sampling trips
monthly for the first two years of the project. Currently there is no
regular program of oceanographic sampling in the SoG, and so these
surveys will provide monthly snapshots of the vertical distribution of
hydrographic, biological and chemical properties (Fig. 1). The second
portion of our field work will involve instrumenting two of the BC
Ferries that travel regularly between Tsawwassen and Nanaimo. Once
established, it is envisioned that this automated sampling program will
run over entire the life of this project, (and hopefully be continued
in the future). Our ferry data will be supplemented by data from a
similar instrument package already in use by Dr. Jim Gower (DFO) along
the southern BC Ferries route through the Gulf Islands (Fig. 1).
Whereas the hovercraft surveys will provide us with monthly snapshots
of the 3D structure of the SoG, the ferry sampling program will provide
high temporal resolution data about the near surface oceanographic
conditions in the southern and central portions of the SoG. Parameters
to be measured from the ferries will include temperature, salinity,
nitrate and fluorescence.
REFERENCES: [1] Beamish et al.
(1999) Can. J. Fish. Aquat. Sci. 56:506-515. [2] Bornhold (2000) M.Sc.
Thesis, UBC. [5] Denman & Pena (1999) Deep-sea Res. II 46:
2877-2908. [5.5] Dery, F. (1992) M.Sc.Thesis, McGill Univ. [7] Fulton
(1973) J. Fish. Res. Board Can. 30:811-815. [8] Gardner (1972) M.Sc.
Thesis, Institute of Oceanography, UBC. [9]Griffin & LeBlond (1990)
Est. Coastal Shelf Sci. 30: 275-297. [10] Harrison et al. (1983) Can.
J. Fish. Aquat. Sci. 40: 1064-1094. [11] Hsieh et al (1995) Can. J.
Fish. Aquat. Sci. 52: 325-334. [12] Ianson & Allen (submitted)
Global. Biogeo. Cycles. [13] Large & Crawford (1995) J. Phys.
Oceanogr. 25: 2831-2852. [14] Large et al. (1994) Rev. Geophys. 32:
363-403. [15] LeBlond (1983) Can. J. Fish. Aquat. Sci. 40: 1033-1063.
[16] Li et al (1999) Atmos.-Ocean 37:1-19. [17] Li et al. (2000) Est.
Coastal Shelf Sci. 50:467-488. [18] Mackas & Harrison (1997) Est.
Coastal Shelf Sci. 44: 1-21. [21] Miller et al. (1984) Prog. Oceanog.
13:201-243. [22] Pawlowicz (in press) Est. Coastal Shelf. Sci. [23]
Pawlowicz & Farmer (1998) J. Geophys. Res. 103: 30695-30711. [24]
Pepin et al. (submitted) Fish. Oceanogr. [24.5] Robert, M. M.Sc.
Thesis, McGill Univ. [25] Rogers (1997) J. Climate 10: 1635-1647.
[27]Stockner et al. (1979) J. Fish. Res. Board Can. 36: 657-666. [28]
Takahashi et al. (1977) Deep Sea Res. 24: 775-780. [29]] Takahashi et
al. (1978) J. Exp. Mar. Biol. Ecol. 31: 283-301. [30] Taylor (1975)
Environ. Lett. 9: 103-119. [31] Walters et al. (1997) Rev. Fish Biol.
& Fisheries 7:139-172. [32] Yin et al. (1996) Mar. Ecol. Prog. Ser.
138: 255-263. [33]Yin et al. (1997a) Can. J. Fish. Aquat. Sci. 54:
1015-1024. [34] Yin et al. (1997b) Mar. Ecol. Prog. Ser. 161: 173-183.
[35] Yin et al. (1997c) Can. J. Fish. Aquat. Sci. 54: 1985-1995.
SECTION 2 - TRAINING OF HIGHLY
QUALIFIED PERSONNEL: We will train at least ten highly qualified
personnel during the four years of this project (2 postdocs, 1
technician, and 7 grad students). Besides those students funded
directly by this proposal, many other students in the Department of
Earth and Ocean Sciences will benefit from interactions with the
multidisciplinary team we are assembling. Five MSc students and two PhD
students will be partially funded through this project. Initial plans
suggest the following topics would be appropriate, but this may change
depending on the students available :
(1) MSc: Interannual variability in Nutrient fluxes and the input into
SoG (with Pawlowicz)
(2) PhD: 3D model of the Strait of Georgia (with Pawlowicz & Li)
(3) MSc: Short and long term variability of physical forcing in the
Strait of Georgia and its relationship to primary production (with
Ingram)
(4) MSc: Physical determination of size-structure of summer
phytoplankton community (with Allen, Denman & Harrison)
(5) PhD: Sensitivity of Neocalanus plumchrus biomass and timing to
interannual variability in temperature & food availability (with
Dower & Allen)
(6) MSc: Impact of El-Nino events on surface temperature and salinity,
and its relationship to primary productivity in SoG (Ingram &
Dower)
(7) MSc: Linking top-down and bottom-up models in the Strait of Georgia
(Dower & Walters)
Students will gain a thorough background in
their main field of study (biological or physical oceanography) in
addtion to developing a broadly based oceanographic skill set (e.g.
field observations and interpretation or modeling and interpretation).
Each will also gain a understanding of the other field and the other
skill set. Belonging to a large project will enable the students to
meet (and have on their supervisor committee) a cross-section of
oceanographers. Our monthly meetings will also ensure that they learn
about and collaborate with the other scientists and students. These
students will be highly trained specialists and be conversant with the
language and tools of a broad field; the emerging hot topic of
biological-physical coupling.
Two postdoctoral fellows will (sequentially) oversee the modeling
effort (i.e. two years each). These young scientists will be trained in
the detailed science of the other field (they will likely be either
physicists or biologists), in coordinating the efforts of a number of
students, in mentoring and leadership, in choosing topics for
scientific papers and focusing research towards "the big questions" and
in grantsmanship. Our plan is that these scientists will leave our
group ready to accept the challenges of an Assistant Professor or a
Research Scientist position.
We will also partially fund one technical person to oversee and
participate in the monthly hovercraft cruise program. This position
will probably go to a recent Masters graduate, who will receive
additional training in field techniques, field organization, leadership
and mentoring (as they aid the students on the cruises), and scientific
liaison.
Of course the other beneficiaries of a large multidisciplinary,
multi-skill project of this sort will be the PI's. Given the breadth of
the proposed research we look forward to learning from each other, and
broadening our own knowledge and skill sets.
SECTION 3 - INTERACTIONS WITH
NON-ACADEMIC ORGANIZATIONS:
Our primary collaborator and partner is Fisheries and Oceans Canada,
and in particular their Ocean Science and Productivity Division (Head:
Robin Brown). Our proposal was designed in concert with their DFO
Strategic Proposal: "Co-variability of Coastal Marine Ecosystems:
Determining the Responses to Environmental Stress and Change within the
Interconnected Basins of Southwestern British Columbia". They see our
proposal as integral (Allen, Dower and Harrison are named as primary
collaborators in their proposal). We will provide the research
component into the biological-physical coupling in the Strait of
Georgia. Their proposal was submitted in December 2000 and has been
funded. Specifically we plan to collaborate with DFO scientists
Angelica Pena, Diane Masson, Richard Thomson, David Mackas, Richard
Beamish, Jim Gower and Ming Li. In addition to comparing model results
(they are modeling the west cast of Vancouver Island while we are
modeling the SoG) we have made plans to co-ordinate our SoG sampling
plans to supplement each others work, but also to prevent unnecessary
(and costly) duplication of effort. Our secondary collaborator is Parks
Canada. Parks Canada is working toward the creation of a marine park in
the Southern Gulf Islands. Understanding the state of the marine
ecosystem in the SoG will be integral to monitoring the biological
health of this Park. We will share our modeling results as well as our
field data from the region with Tom Tomascik and Cliff Robinson, who
will be our direct Parks Canada contacts (Letter of support attached).
Details of collaboration with Fisheries & Oceans Canada:
Angelica Pena is assembling a bio-physical lower trophic level
model for the La Perouse region of the West Coast of Vancouver Island
(WCVI). We (Denman and Allen) plan to actively exchange ideas on the
biological and physical components of our models. In Year 3, both
groups will have determined the basic physical-biological coupling of
there region. We will organize a workshop to analyze why the SoG and
WCVI are out of phase.
Diane Masson and Richard Thomson are leading the ship-board
plankton and physical surveys component and the moored time series
components, respectively, of the Co-variability project. Mooring data
will be collected from the WCVI, SJF, Howe Sound, Jervis Inlet and the
SoG. In the SoG, thermistor chains, three deep current meters and a
bottom-mounted ADCP will measure deep and intermediate water renewal
events. These detailed measurements of the deep/intermediate water
complement the budget approach we will take. Richard Thomson will
directly collaborate with us (Allen) to collect acoustic backscatter
measurements of mesozooplankton (and fish) biomass. One WASP will be
moored in the southern SoG.
David Mackas is collecting broad zooplankton samples from
both the WCVI and inlets around the SoG. Dower and Mackas will
coordinate their zooplankton sampling efforts in the SoG. Mackas will
also provide access to the zooplankton database that his group has
assembled at IOS. This data will be critical in initializing the
Neocalanus life-history model. Richard Beamish has been collecting
fisheries data in the SoG for over 20 years. Beamish has agreed to
provide Dower with access to this data for use in testing the
"heuristic rules" developed from our 1D biological-physical coupled
model. He will also collaborate with us (Walters) in the
construction/comparison of the Ecosim upper-trophic level model for the
SoG. Jim Gower has already instrumented one of the three principal
ferry tracks in the southern SoG. We plan to instrument the other two.
By combining data from all three ferry tracks we will obtain excellent
temporal and spatial coverage of the near surface water properties in
the southern SoG. As satellite data is difficult to interpret in this
region (silt from the water is hard to differentiate from
phytoplankton), this ferry data will be invaluable.
Ming Li will develop a 3D physical model of the SoG/SJF
estuarine system in collaboration with us (Pawlowicz). The model will
be coupled to a simplified biological model. We (Allen, Dower, Denman)
will have detailed exchanges on the choices for the this model based on
our results from the 1D model with complicated biology. Similarly, Li's
results from the 3D model will have direct impact on how spatially
varying physical processes are parameterized in our 1D models (Allen,
Pawlowicz). Li will use sigma-coordinate Princeton Ocean Model (POM) as
the physical model and a three-compartment NPZ model as the biological
model. This model is a natural extension of a coupled box model that Li
et al.(2000) have developed for the Georgia-Fuca estuary. While the 1D
vertical model will focus on the role of wind mixing in phytoplankton
production, the 3D model will be used to investigate horizontal
advection and spatial variability.
SECTION 4 - TRANSFER OF KNOWLEDGE
& BENEFITS TO CANADA: Knowledge & Technology Transfer: We
will develop the knowledge necessary to generate a series of rules that
can be used to help determine "the state of the Strait". The main user
of this information will be our primary partner, Fisheries & Oceans
Canada, in addition to the international oceanographic and fisheries
scientific communities. We will transfer this knowledge to these groups
in a variety of ways.
On a local level, our monthly seminars at UBC will provide a means of
communicating our results to colleagues within the oceanographic and
fisheries community. UBC has a long history of conducting leading-edge
marine science in Canada and we see these monthly meetings as an
important means of getting real-time feedback on our progress from a
wide ranging group of world class marine scientists.
Our closest ties to Fisheries and Oceans will be through their
Strategic Science project "Co-variability of Coastal Marine
Ecosystems", led by Richard Thomson. We will work directly with several
DFO scientists from this program in both our field work (e.g. Thomson,
Masson and Mackas and Gower) and in model development and testing (Li,
Pena and Beamish). We have budgeted funds to enable members of our
group to spend time working side-by-side with our collaborators at both
the Institute of Ocean Sciences (Sidney) and the Pacific Biological
Station (Nanaimo). In addition to those scientists in DFO's
Co-variability project, we will communicate our progress and results to
the wider DFO community by having our PI's give formal scientific
seminars at IOS or PBS at least once a year.
UBC and DFO are both key players in Canada's delegation to PICES, the
North Pacific Marine Science Organization. In recent years, three
members of our team (Allen, Harrison and Denman) have each chaired
special sessions at annual PICES meetings (held alternatively in
Canada, the US, Russia, Korea, China and Japan). We will take advantage
of these strong ties to the Pacific Rim marine science community by
having members of our team chair a Special Session on Coastal Ecosystem
Modeling (at a PICES Meeting in either Year 3 or 4 of our project)
where we can highlight our findings. Additionally, our PI's, graduate
students and postdocs will present results from our research at other
international scientific meetings (e.g. Canadian Conference for
Fisheries Research, Canadian Meteorological and Oceanographic Society,
American Geophysical Union, American Society of Limnology &
Oceanography, and the American Fisheries Society). In terms of
publications, we will submit the results of our work to leading
peer-reviewed scientific journals. As well, given the large field
component in our project, we will compile annual technical data reports
which we will archive with DFO. In Year 4 we will use one of our visits
to DFO to organize a workshop where the results from our models in the
SoG can be compared with DFO's modeling efforts on the west coast of
Vancouver Island.
Benefits to Canada and Non-Academic
Participating Organizations: This research will benefit Canada in two
main ways. Most Canadians are aware that climate change is occurring
and that its effects are already being felt in Canada. Given that
Canada's economic well-being relies heavily on renewable resources
(including marine resources), understanding how such resources may be
affected by climate change in the future is one of the biggest
challenges facing Canadians today. Of course, before we can accurately
predict how these resources may change in the future, it is imperative
that we first understand the factors that govern natural variability in
our renewable marine resources today. By focusing on the processes and
mechanisms linking physics and biology in our natural laboratory, the
SoG, we will gain important insights into how and when marine
ecosystems are sensitive to environmental change and thus help to build
a more informed basis for predicting the effects of climate change in
the future.
The results from this project will be used primarily by Fisheries &
Oceans Canada. In the past, traditional approaches to management and
stock assessment rarely incorporated environmental data, in part
because it was assumed that environmental and ecological conditions do
not change significantly over time. However, we now know that
environments and ecosystems do change on both interannual and
interdecadal time scales. Thus, it has become clear that fisheries
stock assessment studies are not sufficient in themselves to provide an
adequate basis for management decisions and to ensure sustainable
fisheries. In recent years (particularly following the passing of the
Oceans Act in 1997) DFO has moved toward newer strategies rooted in
ideas of "ecosystem management" and "the precautionary approach". Not
surprisingly, the implementation of these new approaches will also
require the development of new tools and strategies. The heuristic
rule-based system that we will develop here will provide a tool that
Fisheries & Oceans can use to incorporate detailed environmental
information into their management plans. Using this system managers
will be able to evaluate the "state of the Strait" in any given year.
By understanding the physical factors that determine the level of
primary and secondary production, it will be possible to classify
individual years as being "better than average", "average", or "less
than average". Such rules will assist managers in making well-informed
decisions about the possible consequences of different management
options. For instance, by knowing that the environmental conditions in
the SoG in a particular spring will most likely have created poor
conditions for plankton production, managers might decide to be more
cautious in their predictions of recruitment of various fish stocks in
that year.
A second benefit will come from the novel approach we propose for
building and testing our models. Far too often, ecosystem modeling is
done in isolation, with only vague connections to real data. In
contrast, we will employ at least three different models (1D coupled
biological-physical models, Ecosim models, and Ming Li's 3D physical
model) and an innovative field program to understand the links between
physics and biology in the Strait. Moreover, we propose to use our
top-down model (e.g. Ecosim) to help test the validity of the "rules"
generated from the 1D coupled biological-physical model. We will
supplement this with tests based on a time-series of fisheries data
collected in the Strait of Georgia over the last 20 years (in
collaboration with R. Beamish). This approach of combining top-down and
bottom-up models (and combining oceanographic and fisheries data) from
the same ecosystem at the same time has rarely, if ever, been
attempted. Thus, Canada will benefit in that the scientists and student
involved in our SoG project will be trained in this new approach, in
the hope that it can applied to other marine ecosystems elsewhere in
Canada.
Fraser River Plume: (Click on
images to enlarge)
Suspended Particles
2000.08.30 from NOAA 14 Satellite Imagery |
SST 2000.08.30 from NOAA 14
Satellite Imagery |
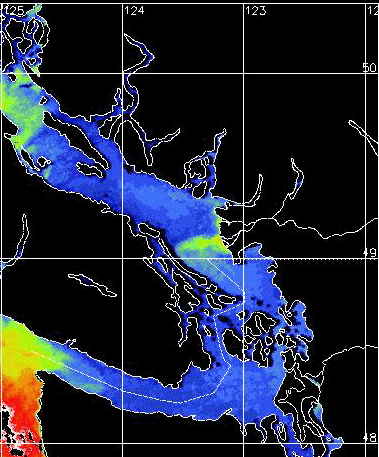 |
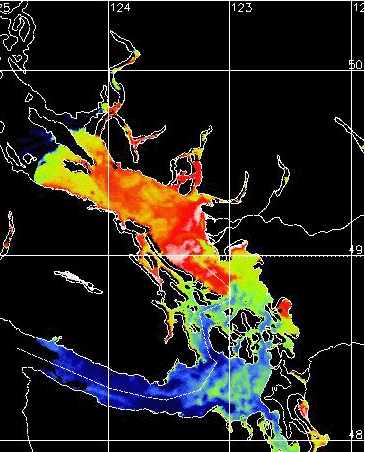 |
[Home] [Science] [Data] [Positions] [Contacts]
[Links]
|